Solutions to quantum computing’s scalability problem are in constant development. Integrated photonics are a valuable tool for researchers to avoid the uncertainties of free space optics. Silicon nitride, with its low optical losses and passive components, allowed researchers in ETH Zurich to implement novel ion traps, new qubit preparation protocols, fine ion control with structured light, and high-purity circular light polarizations. The research contributes significantly towards better scalability and robustness of quantum applications.
Photonic Ion Traps Help Break New Ground in Quantum Processing
In 2020, Nature published a paper by the Trapped Ion Quantum Information group at ETH Zurich featuring a novel ion trap which used integrated photonics to manipulate multiple ions at high fidelity [1]. The integration of light routing on chip made great advancements in the scaling and robustness of the ion trap setup, both necessary improvements for the development of quantum computing. Without free space optics, the system was less vulnerable to vibrations and beam drifting. The ultra-low losses of our TriPleX platform additionally enabled ground-state ion motion cooling, which is essential for multi-qubit logic.
Since then, ETH Zurich have been deploying the photonic integrated circuit (PIC) designs we manufactured for them to great success. Three new papers have been published this year by the group, with different applications.
New Dissipative Engineering Protocol
In Physical Review Letters, the same device deployed in the aforementioned Nature paper was used in a new protocol for qubit entanglement via dissipative engineering [2]. In this new scheme, two qubits can be pumped into a singlet Bell state, where their entanglement is maximized, with a deterministic collective optical pumping process. The method allows for faster convergence, where a separable state comes to have 83(1)% singlet fidelity in 16 pumping cycles, and states with ~70% fidelity reach 93(1)% fidelity in the same duration. This was demonstrated with two trapped ions in the PIC-enabled trap without resorting to ground-state cooling.
The completed ion trap chip with electrical and optical interfaces added by the team at ETH. Photograph: K. Mehta / ETH Zurich
Structured Light For Single Ion Control
Using the same device design, the group also investigated structured light for atomic coupling control [3]. The advantage of using an integrated photonic device for this is, once again, scaling! Free space laser beams become quite impractical when many are required, especially given the small scale at which such spatial manipulation is conducted. Integrated photonics additionally eliminates the necessity for active stabilization of the lasers, as the chips are designed with the ion trap in mind and can reliably reproduce stable interference patterns. Beams propagating into opposing grating outcouplers were used to create an out-of-plane standing wave interference pattern, with which quadrupole transitions were driven in a single calcium ion, 50 µm above trap surface. The relative phase of the beams and the standing wave were passively controlled by the PIC. The use of PIC-enabled structured light in this manner is novel for quantum applications, whereas it has seen more use in other fields.
Illustrations of the structured light ion trap [3]. (a) Layout of the device, including the trap electrodes and the photonic structures. The grating couplers emit 729 nm light out-of-plane to control the ion 50 μm above the chip, represented by the green dot. (b) Schematic of the light field intensity, with an inset for the electronic structure and transition wavelengths (in nm) of the calcium-40 ion.
Pure Circular Polarization with Microring Resonators
Finally, in Applied Physics Letters, the group reported using integrated microring resonators to generate highly pure circularly polarized light [4]. Circular polarization is critical for various methods of atomic systems control, such as laser cooling, selective optical pumping, and closed cycling transitions. Active phase tuning was necessary in previous implementations of circular polarization emitters, which means a constant compromise with emission intensity and inevitable polarization impurities. By introducing an azimuthal gratings to the ring resonator, light can be coupled out of plane of the rings with a circular polarization. The device achieved 17% efficiency (from input to output) without optimising cross-sectional substrate reflection.
The work of the researchers at ETH Zurich highlights the vast possibility space in integrated photonics for quantum applications, much of it unexplored. Creative designs require a versatile platform, adaptable to different applications via broad transparency, low losses, and ease of integration. We are happy to provide researchers with the manufacturing capability and expertise to test their ideas, so their time can be directed to key tasks.
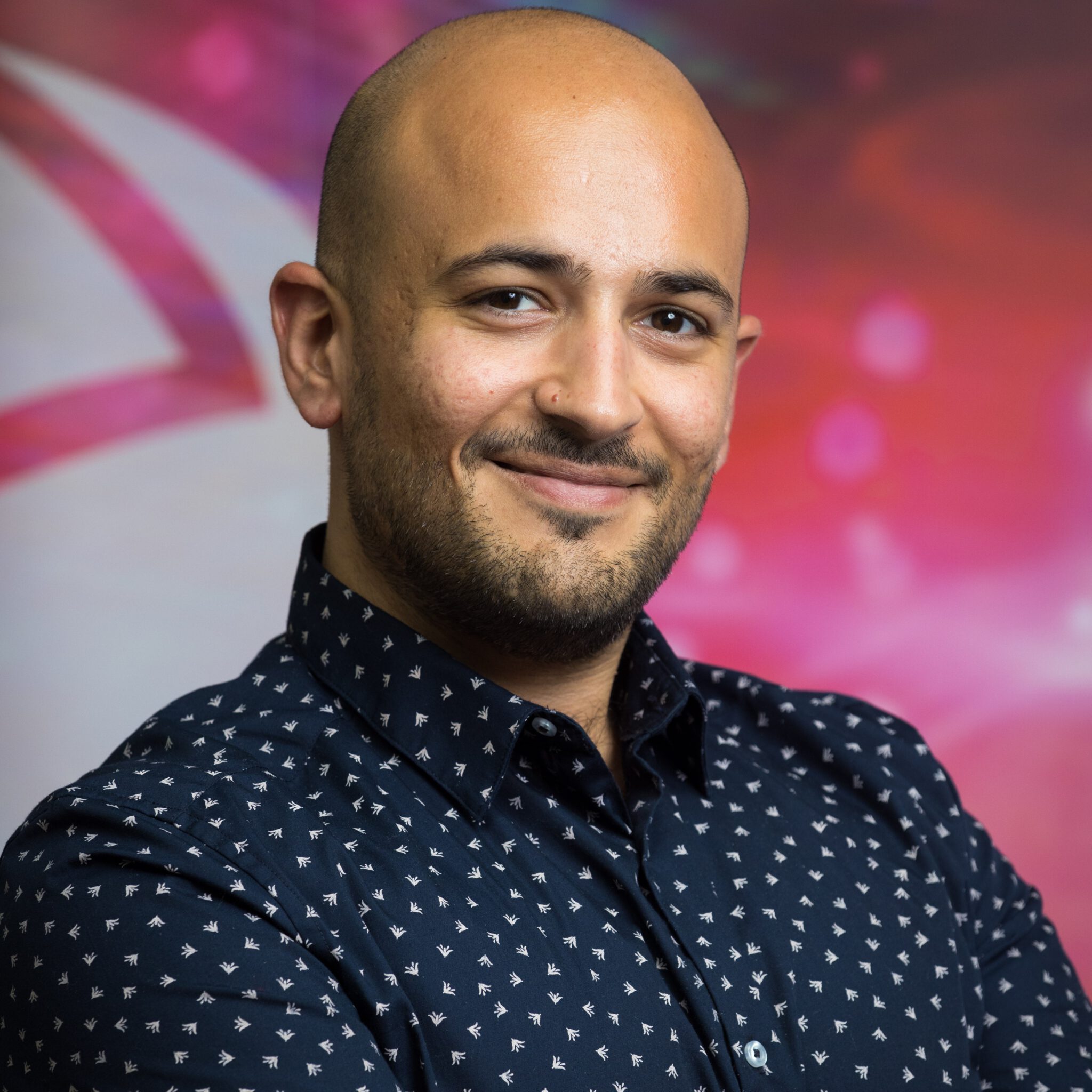
LioniX International’s marketing & communications director, Sadoon graduated with an MSc in Nanotechnology from the University of Twente. When not at an exhibition or meeting industry partners, he can be seen around the offices with a silly hat on.
References
[1] K. K. Mehta, C. Zhang, M. Malinowski, T.-L. Nguyen, M. Stadler, and J. P. Home, ‘Integrated optical multi-ion quantum logic’, Nature, vol. 586, no. 7830, Art. no. 7830, Oct. 2020, DOI: 10.1038/s41586-020-2823-6.
[2] M. Malinowski et al., ‘Generation of a Maximally Entangled State Using Collective Optical Pumping’, Phys. Rev. Lett., vol. 128, no. 8, Feb. 2022, DOI: 10.1103/PhysRevLett.128.080503.
[3] A. R. Vasquez et al., ‘Control of an atomic quadrupole transition in a phase-stable standing wave’, arXiv, Oct. 2022, DOI: 10.48550/arXiv.2210.02597.
[4] L. Massai, T. Schatteburg, J. P. Home, and K. K. Mehta, ‘Pure circularly polarized light emission from waveguide microring resonators’, Appl. Phys. Lett., vol. 121, no. 12, p. 121101, Sep. 2022, DOI: 10.1063/5.0109100.